Evaluating urban intersections: a comparative study of roundabouts versus protected U-turns: which is better?
Guest editor: Nikiforos Stamatiadis, University of Kentucky, the United States of America
Reviewers: Apostolos Anagnostopoulos, Aristotle University of Thessaloniki, Greece
Panayotis Papantoniou, University of West Attica, Greece
Received: 15 June 2024; Accepted: 1 December 2024; Published: 11 January 2025
Abstract
Effective urban traffic management is a significant challenge in rapidly urbanizing areas with increased vehicular usage, impacting both congestion and safety. Busy cities, like many urban centers, face these issues. Roundabouts, known for their ability to reduce severe crashes, represent a traditional approach to intersection design. However, in high-traffic conditions, roundabouts can become less efficient, leading to increased congestion. Alternatively, protected U-turns, which are a relatively modern approach, are designed to handle high traffic volumes more effectively. They potentially reduce waiting times and improve overall traffic flow but may pose different safety and longitudinal challenges. This study aims to explore these complexities by analyzing the effectiveness of roundabouts and protected U-turns in the context of the unique urban landscape of the Ha'il City in Saudi Arabia. PTV VISSIM was used for dynamic traffic simulation, providing a realistic representation of traffic patterns in Ha'il City. Parameters such as vehicle queuing lengths, wait times, and overall intersection capacity are comprehensively evaluated. The simulation settings are adjusted to reflect different traffic densities and patterns, thus ensuring a thorough understanding of the performance of each intersection type under varied conditions. Additionally, various statistical analysis techniques were used to better understand the relationship between the independent and dependent variables. Results showed scenarios of high traffic volume, roundabouts led to longer vehicle queues and increased wait times. On the other hand, protected U-turns exhibited a notable capability to manage high-volume traffic, effectively reducing congestion and improving intersection throughput. This research may contribute to urban traffic management literature, offering insightful guidelines for future intersection design and traffic flow optimization in rapidly urbanizing cities.
Keywords
geometric design, microsimulation, protected U-turn, PTV VISSIM, roundabout
Introduction
Rapid urbanization causes unusual pressure on the transportation infrastructure system, leading to more congested roadways and increased concerns about the environmental impact of these congestions. In addition, these congested roadways increased the probability of crashes and their resulting injuries, which could affect the transportation system reliability and increase cost. The latest urban mobility report by Schrank et al. (2021) indicates that traffic congestion in the USA costs around 190 billion dollars. Traffic congestion is mainly caused by roadway intersections, especially with left-turning vehicles. Therefore, high left-turning traffic requires a protected left-turn movement phase in the signalized intersection phases. However, the protected left-turn phase could cause major delays when the demand for the left-turn is low due to conflict with other phases' movements and the reserved time for the protected left-turn movement. In addition, the protected left-turn movement would increase the traffic signal cycle, which leads to more travel times and delays due to longer waiting times and start-up lost times per phase. As a result, a wide range of geometric designs was used to improve traffic flow and reduce travel time. For example, converting intersections to interchanges would help eliminate traffic conflicts and potentially enhance traffic flow, and reduce travel time, but adopting this solution would be costly. Another alternative geometric design that has gained interest recently is replacing signalized intersections with roundabouts.
The roundabout design aims to minimize travel time and delays and reduce the number of potential conflict points compared to the intersection, potentially leading to a safer intersection. However, roundabouts could become problematic when poorly designed or experienced high traffic volumes, leading to longer delays, queuing, and more conflict points (Saccomanno et al., 2008). Several studies have done a comparative evaluation of the performance of signalized intersections and roundabouts using simulation methods, and none of these studies concluded with a preferred geometric design due to the high variations in designs and driver behaviors (Liu et al., 2020; Owais et al., 2020; Skřivánek Kubíková et al., 2020). The road network structure and the level of traffic demand are the key factors in determining the number of legs on a roundabout (Qin et al., 2011). Roundabouts could be considered as an appropriate option for organizing traffic flow without traffic control signals when having at-grade intersections with four or more legs. However, it must be noted that a high number of roundabout legs could create more conflict points, which potentially leads to increased complexity in managing traffic control in the roundabout (Polders et al., 2015).
Other non-traditional geometric designs to accommodate indirect left turns have been explored. Hummer (1998) suggested several unconventional options that could be considered for designing urban and suburban arterial roadways. A subsequent study by Liu et al. (2007) aimed to study the operational impacts of the indirect driveway left-turn treatments by comparing the delay and travel times for three different driveway left-turn options. These options included direct left turns at driveways, right turns followed by U-turns at downstream median openings, and right turns followed by U-turns at signalized intersections. It was found that vehicles making a right turn followed by a U-turn at a downstream median opening before a signalized intersection experienced similar total travel times to those making a direct left turn at a driveway (Liu et al., 2007). Tabernero and Sayed (2006) introduced the concept of an upstream signalized crossover intersection and then conducted a comparative assessment with a conventional intersection. Their results showed that the upstream signalized crossover could handle heavy left-turn movements while through movements could also maintain acceptable performance. Furthermore, (Sayed et al., 2006) compared the upstream signalized crossover with a similar conventional design under various volume scenarios, and their results suggested that the upstream signalized crossover could manage traffic flow more effectively. A study by El Esawey and Sayed (2007) compared the traffic operation performance of the upstream signalized crossover and crossover displaced left-turn intersections to a conventional intersection and found that both the upstream signalized crossover and crossover displaced left-turn designs result in reduced delays and increased capacity compared to the conventional design.
Previous studies used computer simulation models to assess the traffic performance of unconventional designs. For instance, (Reid & Hummer, 1999) utilized CORSIM to study the traffic performance on an arterial road with five signalized intersections. The study compared the conventional two-way left-turn lane design with two alternative unconventional designs: the median U-turn crossover and the super-street median crossover design. The simulation results showed that the unconventional designs outperformed the conventional intersection in at least one volume scenario. Another approach was done by Anagnostopoulos et al. (2021), who utilized artificial neural networks to accurately predict the impact of driving behaviors and roundabout features on the capacity of roundabouts. VISSIM microsimulation software emerged as a powerful tool for simulating and evaluating the performance of non-conventional designs due to its ability. The simulation software was widely used in previous studies to compare and assess the performance of different non-conventional designs (Anagnostopoulos & Kehagia, 2020; El Esawey & Sayed, 2007; Zhao et al., 2024).
This study aims to assess the traffic performance when replacing a roundabout with protected U-turn configurations. U-turn was first proposed by Pirdavani et al. (2011) to compare it with a signalized intersection based on the travel time, where it was found to produce lower travel time. These protected U-turn facilities are developed on the major roadway on both sides of the roundabout, which means left-turn movement of the minor roadway and on the major roadway will use the U-turn facilities and, through movement, could use the right lanes to continue without interruption. The design of the U-turn area includes a raised island with a curved section to help guide drivers as they make their U-turn or continue along the main roadway. The curved section also helps drivers safely maneuver around slower or stopped vehicles in the deceleration lane, which is for preparing for the U-turn. A dividing island at the entrance ensures that vehicles can safely enter the U-turn area, while a well-protected merging process is in place at the exit. Additionally, an acceleration lane allows U-turn vehicles to safely merge with the traffic on the main roadway. To that end, VISSIM micro simulation models were used to assess the effectiveness of roundabouts versus U-turns. To achieve the objective of this study, several performance measures such as average delay, level of service (LOS), and queue length were utilized as a basis for comparison of alternatives.
Methodology
As mentioned previously, the roundabouts may not perform efficiently when the traffic volume reaches a certain limit. In Ha'il City, Saudi Arabia, there are multiple roundabouts experiencing long queues when the traffic density is high. To resolve this kind of issue, the city decided to partially close the roundabout to efficiently accommodate the traffic. For the purpose of the study, a three-lane roundabout located at King Saud Road in Ha'il City, Saudi Arabia, which is experiencing high volumes, was selected to assess the operational performance. At this specific location, the northbound and southbound will be closed during the holiday seasons to accommodate the traffic traveling in the east and west directions. In addition to the roundabout, two protected U-turn models were developed for this location to compare the effectiveness of roundabouts versus protected U-turns.
PTV VISSIM 2023 was utilized to develop models for roundabouts and protected U-turns. PTV VISSIM 2023 is a microscopic simulation software that models various transportation systems and transport modes, including vehicles, pedestrians, and cyclists, to provide a realistic and detailed representation of traffic dynamics. As a result of VISSIM simulations, the measures of effectiveness (MOEs), such as average delay, level of service (LOS), and queue length, were obtained as a basis for comparison of alternatives. For LOS, LOS A represents the best traffic condition, and LOS F represents the worst traffic flow condition.
Roundabout model
The roundabout model was designed based on the existing geometry obtained from Google Earth Imagery. The characteristics of roundabouts are listed in Table 1. The traffic volumes were collected for each movement from Monday (21 October 2023) to Friday (25 October 2023), a total of five days. The collected data showed that the eastbound/westbound directions had an average daily traffic (ADT) of 76 465 vehicles. The northbound/southbound directions had an average daily traffic of 5 047 vehicles. Moreover, as the collected data indicated, 90% of vehicles were taking the westbound and eastbound routes. Only 10% were taking northbound and southbound. The distribution of the vehicles for the model development followed the same trend. The input vehicles for eastbound/westbound directions were 4 500 vehicles per hour. The input vehicles for northbound/southbound directions were 500 vehicles per hour. The design speed was 62 miles per hour (100 kilometers per hour) according to the posted speed limit. That said, there is a warning sign that says (roundabout ahead) before entering the roundabout.
Variables |
Values |
---|---|
Number of circulating lanes |
3 |
Width of circulating lane |
12 feet |
Inscribed circle diameter |
235 feet |
Number of entrance lanes (major road) |
3 |
Number of exit lanes (major road) |
3 |
Number of entrance lanes (minor road) |
2 |
Number of exit lanes (minor road) |
2 |
Protected U-turn model
Two protected U-turn models were developed based on the existing roundabout geometry. One model was a three-lane road with a two-lane U-turn to match the existing roundabout. The layout of the proposed protected U-turn model is presented in Figure 1. Another model was a two-lane road with a one-lane U-turn to compare to the previously introduced protected U-turn model, as included in Figure 2. The U-turn and curve designs for the protected U-turn model were calculated based on the following equations for the horizontal curve:
where:
L = length of curve; R = radius of curve, which is obtained from Table 3-7 in AASHTO (2018); = angle of curve; D = degree of curve; M = middle ordinance distance; T = length of tangent; LC = long cord, which was obtained from the existing roundabout geometry.
After determining the characteristics of the horizontal curves, the details of protected U-turn designs were refined using AutoCAD and modeled in VISSIM 2023. Additional inputs for VISSIM 2023, such as vehicle inputs, vehicle routes, and reduced speed areas, followed the inputs from the roundabout model. One note needs to be taken that traffic vehicles from the northbound direction were diverged to the adjacent intersection due to the design of the protected U-turn. The northbound direction is closed to traffic.
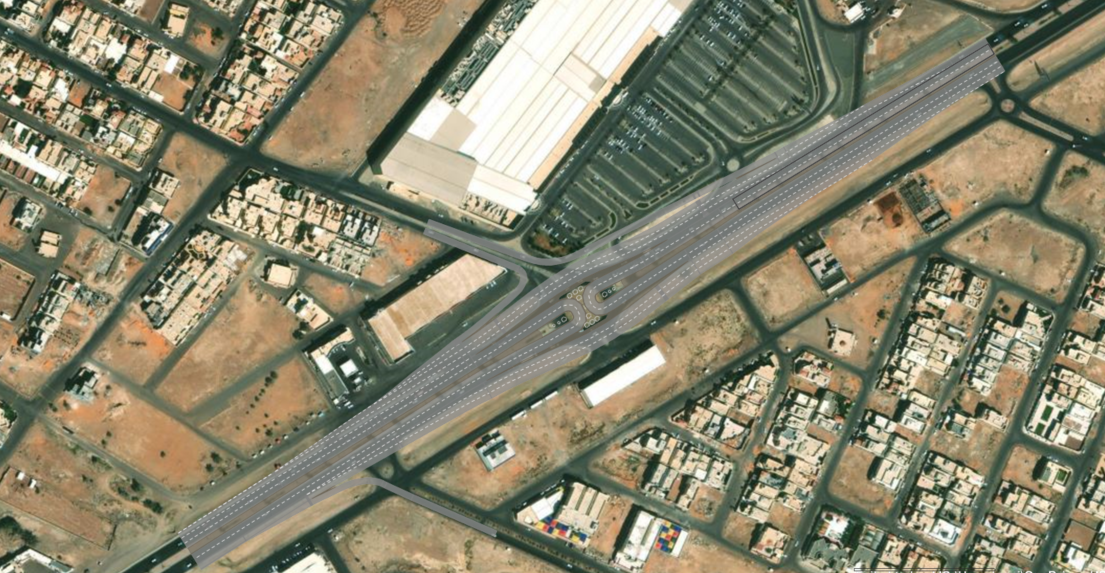
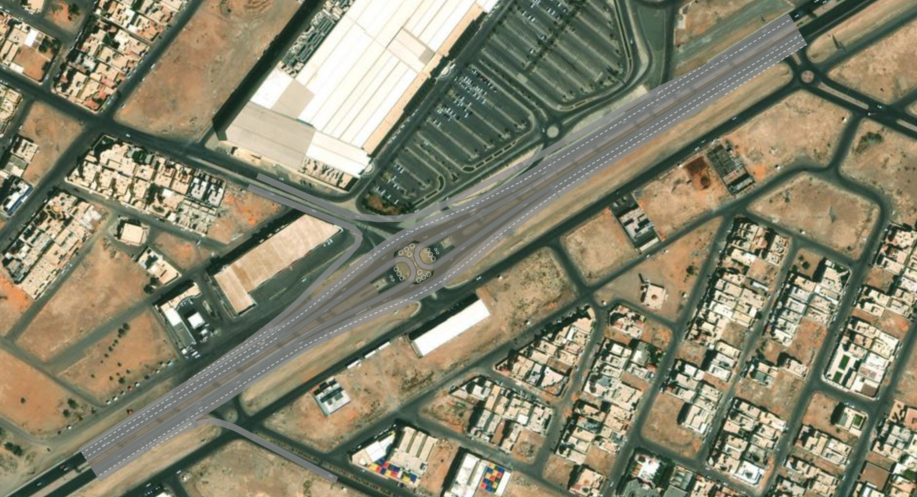
Analysis and results
Table 2 tabulates the input volumes for both roundabout and protected U-turn models. Table 3 presents the VISSIM results for the three-lane roundabout and multi-lane protected U-turn (three-lane road with two-lane U-turn and two-lane road with one-lane U-turn) models. The results indicated that the roundabout operated at LOS F with an average vehicle delay of 84 seconds. The average queue length was about 385 feet. There were 3 232 vehiclespassing this roundabout during 20 to 3 600 seconds.
Model |
E |
W |
N |
S |
---|---|---|---|---|
Three-lane roundabout |
4 500 |
4 500 |
500 |
500 |
Multi-lane protected U-turn |
4 500 |
4 500 |
0 |
500 |
E—eastbound; W—westbound; N—northbound; S—southbound
Results, as presented in Table 3, also show that the three-lane road with a two-lane U-turn operated at LOS A. The average delay was 0 seconds, and the average queue length was 7 feet. A total of 9 029 vehicles would pass this location in 20 to 3 600 seconds. Another model with one lane reduced road and U-turn also operated at LOS A. The average delay was about 0 seconds, and the average queue length was about 14 feet. A total of 8 955 vehicles would pass this location in between 20 to 3 600 seconds. Since both models showed similar performance, the two-lane road with a one-lane U-turn is sufficient for this specific location.
Model |
Vehicle delay (sec) |
Level of service (LOS) |
Queue length (ft) |
Number of vehicles that passed from 20–3 600 sec |
---|---|---|---|---|
Three-lane roundabout |
84.19 |
LOS F |
385.31 |
3 232 |
Three-lane road with two-lane U-turn |
0.00 |
LOS A |
6.83 |
9 029 |
Two-lane road with one-lane U-turn |
0.04 |
LOS A |
14.41 |
8 955 |
Based on the models of roundabouts and two protected U-turn models, the protected U-turn had better performance when compared to the three-lane roundabout. To better understand the performance of a three-lane roundabout, the roundabout model was evaluated with different volumes to determine the limitations of volume that the existing three-lane roundabout is capable of operating. The distribution of the volumes followed the distribution percentage described in the previous section, which was 90% of vehicles traveling in eastbound/westbound directions, while 10% of vehicles traveling in northbound/southbound directions. Table 4 lists the results of roundabout performance with different volumes.
Volume |
Vehicle delay (sec) |
Level of service (LOS) |
Queue length (ft) |
Number of vehicles that passed from 20–3 600 sec |
|
---|---|---|---|---|---|
eastbound / westbound |
northbound / southbound |
||||
500 |
60 |
0.06 |
LOS A |
15.16 |
1 143 |
600 |
70 |
0.12 |
LOS A |
16.63 |
1 331 |
700 |
80 |
0.20 |
LOS A |
18.04 |
1 507 |
800 |
90 |
0.30 |
LOS A |
20.29 |
1 702 |
900 |
100 |
0.45 |
LOS A |
22.38 |
1 943 |
1 000 |
110 |
0.70 |
LOS A |
24.71 |
2 147 |
1 100 |
120 |
1.09 |
LOS A |
28.25 |
2 344 |
1 200 |
130 |
1.48 |
LOS A |
30.84 |
2 573 |
1 300 |
140 |
3.28 |
LOS B |
41.13 |
2 802 |
1 400 |
150 |
7.63 |
LOS C |
64.10 |
2 995 |
1 500 |
160 |
11.69 |
LOS D |
82.81 |
3 202 |
1 600 |
170 |
37.28 |
LOS F |
192.80 |
3 149 |
1 700 |
180 |
44.57 |
LOS F |
225.45 |
3 162 |
As is shown in Table 4, the volume started at 500 vehicles per hour with 100 vehicles per hour increment for the eastbound/westbound directions and 60 vehicles per hour with 10 vehicles per hour increment for the northbound/southbound directions. The average delays, queue length, and number of vehicles passing this location between 20 to 3 600 seconds increased as the volumes increased. When the volumes were below 1 400 vehicles per hour and 150 vehicles per hour, the three-lane roundabout had at least LOS C or better. The roundabouts operated at LOS D with 1 500 vehicles per hour and 160 vehicles per hour. Once the volumes reached 1 600 vehicles per hour and 170 vehicles per hour, the roundabout had LOS F. The number of vehicles passing this location between 20 to 3 600 seconds also started to decrease.
Roundabout versus protected U-turn
To better understand the comparison of both treatments, the results were graphically presented in Figure 3. Figure 3a illustrates the relationship between traffic volume and queue length in feet for both roundabouts and protected U-turns. The data reveals a clear contrast in performance under varying traffic conditions. The roundabout demonstrates a significant increase in queue length as traffic volume increases, reaching up to 385 feet at a volume of 4 500 vehicles per hour. In contrast, the protected U-turns, both the three-lane and two-lane road configurations, maintain very low queue lengths even at high traffic volumes. This suggests that protected U-turns are significantly more effective in managing traffic flow and preventing congestion compared to traditional roundabouts. The visual comparison highlights the better capacity of protected U-turns to handle higher traffic volumes without substantial increases in queue length.
Similarly, Figure 3b presents the relationship between traffic volume and vehicle delay in seconds for roundabouts and protected U-turns. The roundabout exhibits a steep increase in vehicle delays as traffic volume increases, with delays reaching over 80 seconds at 4 500 vehicles per hour. On the other hand, the protected U-turn designs show low delays, with the three-lane road maintaining a constant delay of around 0 seconds and the two-lane road showing a slight increase to 0.4 seconds at 4 500 vehicles per hour. This obvious difference in vehicle delay further emphasizes the efficiency of protected U-turns in maintaining smoother traffic flow and reducing wait times, making them a more suitable option for high-traffic volumes in urban areas compared to roundabouts.
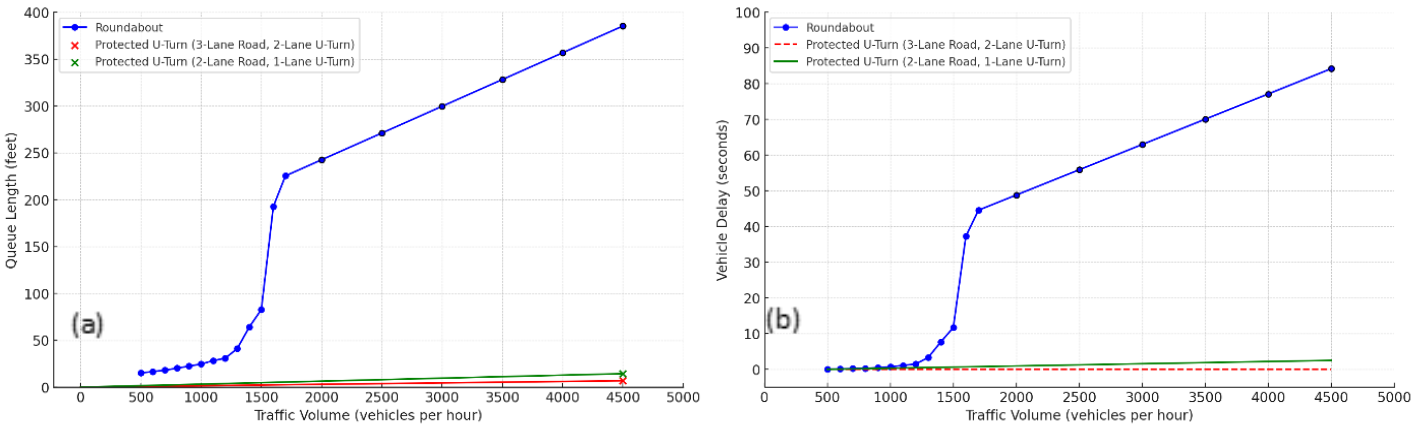
Discussion
The findings of this study offer significant insight regarding the performance of roundabouts and protected U-turns in urban contexts, particularly in Ha'il City, Saudi Arabia. Micro traffic simulation using PTV VISSIM analysis showed that protected U-turns are more effective than roundabouts in managing high-traffic volume scenarios and congestion reduction; thus, better traffic flow is maintained. As such, the results of this research align with other studies, including (Pirdavani et al., 2011), showing that protected U-turn facilities are better at minimizing delay times than conventional signalized intersections.
For high-traffic volume scenarios, it was observed that roundabouts were found to lead to longer vehicle queues and increased wait times, confirming the concerns presented by Saccomanno et al. (2008) regarding the limitations of roundabouts under heavy traffic conditions. However, the protected U-turns showed a capability to handle high traffic volumes effectively. The simulations showed that the protected U-turns had effective ways of managing the traffic with minimal delays and shorter queue lengths by providing better intersection throughput and a reduction of congestion. This performance was achieved due, perhaps, to the operations supported by the design features of the protected U-turns, such as raised islands and well-separated merging processes, ensuring safer and more efficient traffic movements.
The critical implications of this study relate to urban traffic management in fast-growing cities. Protected U-turns have the potential to provide a proper solution to high traffic volume when compared to roundabouts, specifically for cities experiencing rapid growth rates. The results suggest that city planners and traffic engineers may apply this design in certain high-traffic urban areas to improve traffic flow and reduce congestion. Moreover, the assessment of performances of the designed roadway configurations using PTV VISSIM software for dynamic traffic simulation has proven to be quite practical. The software has the ability to model various transportation systems and modes of operation; for example, it gave realistic scenarios of traffic operations in this study.
The measures of effectiveness (MOEs), such as average delay, level of service (LOS), and queue length, served as crucial indicators for comparing the alternatives. The roundabout model, operating at LOS F with an average vehicle delay of 84 seconds and an average queue length of 385 feet, was clearly outperformed by the protected U-turn models, both of which operated at LOS A with significantly lower delays and queue lengths. The implications of such findings can be beneficial and extended beyond this case analysis. They may even be applied at more significant levels concerning urban traffic management strategies to meet the challenges of innovative designs at intersections posed by rapid urbanization. Future research could explore long-term impacts on traffic safety and environmental factors as part of a holistic view of benefits and potential drawbacks associated with protected U-turns.
Conclusions
This study provides a comprehensive comparative evaluation of roundabouts versus protected U-turns, showing how effective the performance of protected U-turns was in managing high traffic volumes in urban settings. The findings highlight the potential of protected U-turns to significantly reduce congestion, improve traffic flow, and enhance intersection capacity. Thus making them a viable alternative to traditional roundabouts, particularly in rapidly urbanizing areas. The use of PTV VISSIM for dynamic traffic simulation was proven to be effective in demonstrating a realistic and detailed analysis of intersection performance. This could provide a valuable tool for city planners and traffic engineers in assessing different roadway configurations. To that end, the results suggest that implementing protected U-turns can lead to notable improvements in urban traffic management. Future research should focus on the long-term impacts of protected U-turns on traffic safety and environmental sustainability, providing a more holistic view of their benefits and potential failure mechanisms. Additionally, examining the influence of emerging technologies such as autonomous vehicles and the economic implications could also play a role in deciding the effectiveness of these treatments.
Despite the promising results, this study, like other studies, has several limitations. For example, the simulation models used in PTV VISSIM are high-level and preliminarily developed and validated based on specific traffic conditions and geometric configurations of Ha'il City, Saudi Arabia. These conditions may not be fully extended to other urban environments with different traffic patterns, driver behaviors, and road geometries. However, future studies should consider a broader range of urban settings to calibrate the models and validate the applicability of these findings across different contexts. Moreover, while the study focuses on traffic performance metrics such as average delay, level of service (LOS), and queue length, it does not extensively address the safety implications of protected U-turns compared to roundabouts. Although the design of protected U-turns aims to improve traffic flow, potential safety concerns, such as increased conflict points or crash rates, were not investigated. Future research should incorporate safety analysis to provide a comprehensive evaluation of these alternative designs. Furthermore, this study specifically focuses on three-lane roundabouts. In contrast, one-lane or two-lane roundabouts are more commonly found in other urban areas due to space constraints. Given their prevalence, it will be important for future research to investigate the performance and characteristics of one-lane and two-lane roundabouts to provide a more comprehensive understanding of roundabout design and operation across different urban settings. Finally, the study did not account for environmental impacts such as emissions and fuel consumption, which are crucial considerations in urban traffic management. Evaluating the environmental benefits or the problems that could be raised by protected U-turns compared to roundabouts would provide a more holistic view of their sustainability. For example, a study could integrate environmental performance metrics to assess the overall impact of different intersection designs on urban ecosystems. With that said, while this study provides valuable insights into the comparative performance of roundabouts and protected U-turns, further research is needed to address its limitations and explore additional dimensions of urban traffic management.
Declaration of competing interests
The authors report no competing interests.
Ethics statement
The methods for data collection in the present study have been approved by the University of Ha'il and Ha’il Municipality.
Funding
This work has been funded by the University of Ha'il and Ha’il Municipality.
Declaration of generative AI use in writing
The authors declare that no generative AI was used in this work.
CRediT contribution statement
Nawaf Alnawmasi: Conceptualization, Data curation, Investigation, Methodology, Software, Visualization, Writing—original draft.
Hisham Jashami: Conceptualization, Data curation, Investigation, Methodology, Software, Visualization, Writing—original draft.
Qiuqi Cai: Conceptualization, Data curation, Investigation, Methodology, Software, Visualization, Writing—original draft.