Enhancing cyclist safety in cyclist-vehicle interactions through early hazard notifications: a comparison of bi-modal cues at head level
Guest editor: Ragnhild Davidse, SWOV Institute for Road Safety Research, the Netherlands
Reviewers: Reinier Jansen, SWOV Institute for Road Safety Research, the Netherlands
Willem Vlakveld, SWOV Institute for Road Safety Research, the Netherlands
Received: 30 January 2024; Accepted: 7 October 2024; Published: 13 November 2024
Abstract
Cyclists frequently face numerous hazards on the road. Often those hazards are posed by motorised vehicles. Advanced support systems that alert cyclists to potential dangers could enhance their safety. However, research in this area, particularly regarding hazard notifications for cyclists, remains sparse. This work assesses bi-modal early hazard notification concepts (combining visual cues with either auditory or tactile feedback) provided at head level (smart glasses with speakers, tactile headband). They are detailing the nature of the hazard, its direction relative to the cyclist, and the timing of exposure. This work investigates cyclists' preference and perception of the proposed concepts for two hazardous situations originating from interactions with vehicles: ‘dooring’, the hazard of a potential collision with an opening door of a parked vehicle (evaluated through a test track study, N = 32) and ‘being overtaken’ which poses the hazard of being cut off or hit by the overtaking vehicle (assessed in a bicycle simulator study, N = 21). The study involved comparisons of supported and unsupported rides, focusing on their impact on usability, intuitiveness, workload, and perceived safety. Our findings reveal varied preferences for the supporting feedback modality, with 56% favouring visual-auditory and 31% visual-tactile. The participants rated user experience, intuitiveness and perceived safety for the use of both concepts quite high. Further, the workload for assisted rides was rated as equally low as for unassisted rides.
Keywords
accident prevention, augmented reality, bi-modal notification, connected traffic, cyclist safety, hazard notifications, head-mounted display, vulnerable road users
Introduction
Cycling is a healthy and sustainable mode of transport. It is also often a faster way to commute in cities than by car or public transport (Pucher & Buehler, 2017). However, accidents remain a significant concern, particularly for vulnerable road users (VRUs) like cyclists. Collisions with motorised vehicles often result in severe or fatal injuries for cyclists (Vissers et al., 2016). Although all road users should exercise caution so as not to harm anyone, vigilance and foresight are essential to stay safe in traffic as others may not always behave correctly. In particular, VRUs could be overlooked by other road users and therefore must continuously identify and evaluate potential hazards, a task that can be particularly challenging with faster moving vehicles or hazards outside their field of vision. This work proposes an early hazard notification system for cyclists to support them in noticing potential traffic hazards.
Hazards or risk factors in traffic can be situations or conditions that potentially cause harm to road users (WHO, 2023). These include hazards from vehicles (e.g. through faulty components), by road users (e.g. incorrect behaviour, errors), from road infrastructure (e.g. inadequate facilities), road conditions (e.g. potholes) or weather conditions (e.g. heavy rain). In the remainder of this article, we exclusively refer to hazards posed by the dangerous behaviour of road users, namely motorists and their passengers.
As an enabling technology for early notifications, we see future connected and intelligent transportation systems (C-ITSs) (Jenkins et al., 2017) that could provide road users with information about their surroundings. Our approach informs cyclists of a detected potential hazard at head level using an augmented reality head-up display (AR-HUD), such as smart cycling glasses (Engo, 2024). This paper presents two user studies we conducted to investigate how potential users perceive bi-modal Hazard Notification concepts in contrast to receiving no feedback (baseline, B). Visual cues indicated (i) the type of hazard, (ii) its relative direction to the cyclist and (iii) the time gap to the hazard. They were combined with auditory (V+A) or tactile (V+T) cues to guide the cyclist and reduce distraction from repeatedly checking the visual display.
In the context of hazard notifications for cyclists, Engbers et al. (2018) investigated front- and rear-view assistance. They found that their participants regarded both assistants positively (usefulness and satisfaction) but notification about front-facing hazards, such as oncoming cyclists, were perceived ‘more as a system confirming what [the] participants could notice themselves’ (Engbers et al., 2018). Notifications about hazards approaching from the behind were considered more useful. Building on these findings, we have examined our notification approaches for two kinds of Hazards that are particularly difficult to detect as the vehicle or the vehicle occupants are out of sight: (i) ‘dooring’ hazards, where cyclists may collide with an opening vehicle door when occupants exit, and (ii) the hazard of ‘being overtaken’, where vehicles could overtake with insufficient lateral distance and could cut off or collide with the cyclist. While drivers ideally should maintain a distance of 1–1.5 meters while overtaking (Dozza et al., 2016; Rasch et al., 2020), insufficient overtaking distance is a common issue (Rasch et al., 2020; Rubie et al., 2020). Early hazard notifications could enable cyclists to react earlier and more controlled in potential dooring situations (braking or swerving) compared to receiving no information about the hazard. In overtaking situations, cyclists could alert motorists to their presence or adjust their position on the lane so that they have sufficient space for evasive action.
In our view, an early hazard notification can be a valuable tool for reducing cyclists’ involvement in accidents by enabling proactive responses. It should be noted that this support is not intended to shift the responsibility for preventing accidents with motorised vehicles onto cyclists but to aid them in noticing hazards. For mutual awareness of hazards, other road users should also be provided with such systems (Jin et al., 2021; Manz et al., 2023). However, our study only focuses on the cyclists’ side.
This work contributes an approach to improving cyclist safety: (i) an early hazard notification concept that (ii) uses bi-modal cues, (iii) provided through a head-mounted device, (iv) to make cyclists aware of potential hazards (v) posed by motorists/their passengers.
Related work
In recent years, academia, industry, and municipalities increasingly focused on ways to make traffic participation safer. As a result of these efforts, for example in Europe, the relative number of road fatalities, for pedestrians (-19%), motorcyclists (-20%), and car passengers (-24%) has decreased between 2010 and 2018; however, for cyclists the numbers stagnated (ETSC, 2020). Additionally, the number of accidents with severe injuries for cyclists is slowly increasing (EU, 2021). Consequently, ways must be found to reduce cyclist accident rates.
External measures to improve road safety for cyclists
One measure to improve road safety for VRUs is infrastructure changes, such as dedicated lanes to separate motorised and non-motorised vehicles (Petegem et al., 2021). Another possible measure would be safer roads through safer vehicles. With built-in sensors, vehicles could sense their surroundings. They could be used, for example, to support drivers in overtaking cyclists (Brijs et al., 2022; Calvi et al., 2021) or to prevent dooring situations (Zhu et al., 2019). Through future C-ITSs that rely on technologies such as vehicle-to-everything (V2X) or others, information about hazards could be obtained. This data could also be used to improve road safety (Bengler et al., 2014).
Hazard notifications for cyclists
In recent years, the interest in directly supporting cyclists has grown (Savino et al., 2021; Schneeberger et al., 2023; von Sawitzky et al., 2021). Beyond assistance in hazard detection, such systems could aid cyclists in, navigation (Albrecht et al., 2016; Huxtable et al., 2014; Matviienko et al., 2019; Savino et al., 2020), exploration (Krauß et al., 2021; Poppinga et al., 2009), group cycling (Kräuter et al., 2016), getting on/off the bike and balancing while cycling (Dubbeldam et al., 2017) or for traffic education of child cyclists (Matviienko et al., 2020).
Although research on cyclist hazard notification is still sparse, various approaches have been proposed and investigated. These include encounters with crossing road users at intersections (Jin et al., 2021; Matviienko et al., 2018; Oczko et al., 2020; Prati et al., 2018; Strohaeker et al., 2022; von Sawitzky et al., 2020), overtaking vehicles (Brummelen et al., 2016; Engbers et al., 2018; Garmin Ltd, 2024), or potential dooring situations (Manz et al., 2023; Sawitzky et al., 2022; Springer-Teumer et al., 2023). Study participants perceived the use of support systems positively and noticed an increase in perceived safety (Manz et al., 2023; Springer-Teumer et al., 2023; von Sawitzky et al., 2022) and found the support to be intuitive and easy to use (Jin et al., 2021; von Sawitzky et al., 2022). Some studies measured reaction time or braking impact, and observed shorter braking times (Prati et al., 2018; Strohaeker et al., 2022).
Integral components of cyclist hazard notifications
We have analysed the proposed concepts of existing work on cyclist hazard notification (listed in Table A.1 in the appendix) by focusing on the key aspects that we see as integral for describing and replicating them:
-
Hazard detection. Assumptions about how hazards are detected.
-
Notification timing. When a notification will be issued.
-
Notification content. The information types used to indicate a hazard.
-
Feedback modality. Visual (V), auditory (A), or tactile (T) in uni- or multi-modal setups.
-
Notification presentation. Patterns and devices used to deliver the content.
Hazard detection
Hazards can be detected by various means, such as on-bike sensors, e.g. radar (Engbers et al., 2018; Garmin Ltd, 2024; Kalaiselvan, 2021; Lindström et al., 2019) or by acoustic ranging (Jin et al., 2021). Another option would be to use cloud services linked to an accident database to indicate accident black spots (Trösterer et al., 2022). Further, future technologies enabling C-ITS (Hernandez-Jayo et al., 2015; Scholliers et al., 2014; Scholliers et al., 2017; Silla et al., 2017) will create opportunities to provide safety-related information to road users. Data obtained could be used, for example, to support cyclists during crossing decisions at intersections (Matviienko et al., 2019; Oczko et al., 2020; von Sawitzky et al., 2020).
Notification timing
Many on-bike sensor approaches issued notifications 4–5 s before a potential collision (Brummelen et al., 2016; Jin et al., 2021; Kalaiselvan, 2021). The system in (Engbers et al., 2018) provided warnings 3 s prior to a hazard. Assuming that C-ITS could theoretically enable earlier notification, they considered quite short timings of 3 s in advance (Oczko et al., 2020; Strohaeker et al., 2022). Such short notices leave cyclists with limited options, such as abrupt braking or abrupt avoidance manoeuvres, e.g. as observed in (Strohaeker et al., 2022) study based on cycling behaviour data. An earlier notification time of 9 s prior was used by (von Sawitzky et al., 2022), inspired by ISO 18682 referring to in-vehicle hazard notifications, which can be classified as warnings (1–3 s before an imminent hazard) or as awareness messages (AMs, 3–10 s before a potential hazard). About 64% of their participants found this early notification appropriate for dooring hazards, while 23% would prefer slightly later notification.
Notification content
Some approaches indicated a hazard without providing further information (Erdei et al., 2021; Jin et al., 2021; Manz et al., 2023; Prati et al., 2018; Strohaeker et al., 2022; Trösterer et al., 2022). Most approaches notified only about one hazard; four addressed multiple hazards (Engbers et al., 2018; Matviienko et al., 2018; Springer-Teumer et al., 2023; Strohaeker et al., 2022). Two systems specified which hazard to anticipate (Garmin Ltd, 2024; von Sawitzky et al., 2021). Both provided early notifications, which allow cyclist to notice and process the additional information. Several concepts included directional information (Brummelen et al., 2016; Engbers et al., 2018; Garmin Ltd, 2024; Matviienko et al., 2018; Oczko et al., 2020; Springer-Teumer et al., 2023; Vo et al., 2021). We assume that directional cues could facilitate the recognition and processing of potential hazards. Additional distance information was considered byvon Sawitzky et al. (2022). Some of their participants reported difficulties in mapping the indicated distance to accurately locate a parked vehicle. We assume that while distance information may be useful for static hazards, e.g. a parked vehicle, distance information may become ambiguous and add complexity when the hazard is in motion, e.g. a vehicle approaching from behind. Vo et al. (2021) used tactile cues to encode distances (far and close). Time information to indicate the approach of/to a potential hazard was suggested byvon Sawitzky et al. (2022), which in our view may be easier to understand than distance cues, as they indicate how quickly a hazard is approaching. Additionally, Matviienko et al. (2022) and von Sawitzky et al. (2020) provided further visual cues to aid in crossing decisions.
Feedback modality
Participants in the studies by Oczko et al. (2020), Trösterer et al. (2022), and (Sawitzky et al., 2022) did not prefer the use of uni-modal visual feedback alone over the alternative cues investigated. Oczko et al. (2020) found that visual-only cues led to significantly more collisions than auditory or haptic cues. Their participants rated the latter two cues as more effective in preventing collisions but perceived haptic feedback as easier to understand, less distracting, and this type of feedback also resulted in shorter reaction times. Trösterer et al. (2022) reported that visual-only cues on cycling glasses were difficult for their participants to perceive, e.g. in bright light conditions. Additional auditory cues could help to mitigate this problem. The analysis of cycling behaviour by (Sawitzky et al., 2022) suggested that uni-modal visual cues on cycling glasses led to similar evasive manoeuvres as with multi-modal visual and auditory cues. However, user experience ratings and follow-up interviews revealed a preference for a combination of visual and auditory cues. Some approaches that supported cyclists at intersections solely investigated visual augmented reality (AR) overlays (Matviienko et al., 2022; von Sawitzky et al., 2020). It was not reported whether participants disliked receiving only visual cues. However, participants of von Sawitzky et al. (2020) stated that an additional acoustic signal would improve the noticeability of the overlay. Visual cues alone could easily be perceived as distracting (Erdei et al., 2021; Matviienko et al., 2019), mainly because cyclists rely heavily on the visual (Mäkelä et al., 2015) channel. Also the auditory channel is required to detect or localize road users (Stelling-Kończak et al., 2016), e.g. to hear approaching vehicles. Therefore, some approaches evaluated only tactile cues (Brummelen et al., 2016; Engbers et al., 2018; Manz et al., 2023; Vo et al., 2021). For (Erdei et al., 2021)'s participants, auditory cues had a higher urgency than tactile or visual cues. Their participants also had difficulty perceiving/noticing visual cues, or perceived them with a delay. Auditory and tactile cues were perceived as equally comfortable and more comfortable than visual cues. The preferred uni-modal feedback was auditory (about 60%) or tactile (about 40%). However, 38% felt uncomfortable using auditory cues if others nearby could hear them. Strohaeker et al. (2022) suggested that combining the two modalities could mitigate missing cues due to ambient noise or ground vibration.
Few studies have explored multimodal hazard notification for cyclists. (Matviienko et al., 2019) examined combining (i) uni-modal cues (visual, auditory or tactile) indicating the direction of a potential hazard in combination with (ii) bi- or tri-modal feedback (combinations of auditory, tactile and visual cues) indicating imminent danger. They found multimodal signals conveyed higher urgency, which we see suitable to convey warnings. However, the combination of visual directional cues and a bi-modal warning indication which also included visual feedback were more distracting. Tri-modal cues were the preferred combination to warn about an imminent hazard. Springer-Teumer et al. (2023) also evaluated a tri-modal approach. Other studies focused on visual and auditory feedback combinations (Garmin Ltd, 2024; Prati et al., 2018; Trösterer et al., 2022; von Sawitzky et al., 2022). The participants in (von Sawitzky et al., 2022)preferred visual cues combined with auditory cues over visual cues alone. Trösterer et al. (2022) reported that their participants preferred auditory feedback alone rather than in combination with visual cues or visual cues alone. They also mentioned visibility problems in bright light conditions, which might have influenced their preference.
Notification presentation
The patterns and intensities of the signals varied between approaches. If patterns were described, we listed them in Table 4 in the appendix. Tactile cues were provided via handlebar grips (Brummelen et al., 2016; Erdei et al., 2021). Engbers et al. (2018) additionally used tactile feedback on the saddle. Vo et al. (2021) explored integrating tactile feedback into cycling helmets. Auditory cues were mostly provided by smartphones mounted on the handlebars (Strohaeker et al., 2022). The emitted sounds may be perceivable by others in the vicinity which may be uncomfortable for the user (Erdei et al., 2021). Alternatively, directional loudspeakers at ear level (Matviienko et al., 2019; von Sawitzky et al., 2022) or bone conduction headphones (Trösterer et al., 2022) have been suggested that offer more private and comfortable feedback. While mostly beeps were used (Erdei et al., 2021; Matviienko et al., 2019; Strohaeker et al., 2022; von Sawitzky et al., 2022), voice messages were also deemed helpful (von Sawitzky et al., 2022), albeit potentially distracting. Visual cues were presented through lights on the handlebar (Oczko et al., 2020), smartphones or other displays (Garmin Ltd, 2024), and smart glasses (Matviienko et al., 2022; Trösterer et al., 2022; von Sawitzky et al., 2020; von Sawitzky et al., 2022). Delivering visual information at head level, e.g. via head-mounted displays, may reduce distraction compared to handlebar-mounted displays, allowing users to obtain them at a glance or from their peripheral view (Matviienko et al., 2019). Smart glasses may also facilitate perception of the visual cue (Erdei et al., 2021; Strohaeker et al., 2022).
Concept for the design of early hazard notification
Below, we outline our design considerations (which partly rely on the works outlined in the previous section) and our notification design. We assumed that usable hazard notifications for cyclists should meet several key requirements. They should inform cyclists early about hazards that may occur in the short term, so that they can take deliberate (proactive) action to avoid a collision. It is also necessary that the information is easily accessible in terms of modality, positioning, and presentation, allowing cyclists to quickly perceive the notification. The communication of the message content should be clear and intuitive so that the cyclist can easily understand the cues. In addition, these cues should not distract the cyclist from the primary task of cycling. Further, they should have a positive impact on situational awareness and cycling behaviour in hazardous situations. Ultimately, the goal is to help cyclists navigate safely through traffic by enabling them to anticipate and avoid hazards.
Design considerations
For hazard detection, we assumed the availability of information via C-ITS. While approaching vehicles could be detected with on-bike sensors, this is not the case for detecting parked and still occupied vehicles. To generate notifications about potential hazards, possible interactions with other road users must first be identified to predict hazards. For this, we assume that C-ITS has access to GPS information (position, heading, speed) and information on whether parked vehicles are occupied (and on which side), as well as a digital twin of the road network (Wang et al., 2022) which will be used to predict only relevant interactions.
For the timing of notifications, we see it crucial that cyclists have adequate time to perceive, comprehend and react to potential hazards. A similar need for an adequate time frame is given in the context of take-over requests in partially automated vehicles where the driver needs to get back into the loop to take over vehicle control. Gold et al. (2013) reported that approximately 7 s are required to (i) perceive an incoming message, (ii) become aware of the current situation, (iii) consider possible actions, and (iv) react accordingly. Despite cyclists’ continuous involvement in cycling compared to the drivers’, we assume a similar time frame is necessary in such a safety-critical situation. Therefore, we decided to consider a notification time of 9 s, as proposed in (Sawitzky et al., 2022) and utilise AMs. The time information of the notification can be understood as time to collision (TTC) until the predicted hazard area.
In our view, the notification content should be targeted information that allows cyclists to understand the situation fast and not add to cognitive load. The correct hazard placement could be supported through directional information to help cyclists quickly locate potential hazards (Engbers et al., 2018; Matviienko et al., 2018; Springer-Teumer et al., 2023). In our view, once a system supports multiple hazards, it also becomes important to specify the kind of hazard. Otherwise, users would have to determine which specific hazard they are notified about. Rather than spending time trying to figure out which hazard was detected, it may be more appropriate to use this time to prepare for taking action. Given our intention to provide early notification of hazards, additional time-based information on when a hazard is expected to be closest could be helpful (Sawitzky et al., 2022). Therefore, in our design concept, we considered information about (i) the type of hazard, (ii) where it is located, and (iii) when it will be closest.
To provide this information, we had to select which notification modalities to employ. When considering notifications about different hazards, we see visual information provided as symbols as an appropriate way to distinguish between kinds of hazards. We adopted a bi-modal approach to counteract the distraction associated with visual cues due to frequent glancing at the display (Erdei et al., 2021). We integrated a second modality to inform the cyclist of an incoming message and to provide guiding cues throughout the notification. As opinions differ on whether tactile or auditory cues are better suited, we investigated each in combination with visual cues. Nees and Walker (2011) highlighted that sound detection, discriminability, and identifiability are key considerations in the design of auditory interfaces; we assumed these factors similarly apply to tactile interfaces.
To minimise distraction and ensure ease of perception, we opted for feedback at head level through smart glasses to deliver visual cues instead of handlebar mounted displays for the presentation of notifications. We assumed that perceiving visual information at head level has a similar effect as head-up displays in cars, which reduce driver distraction away from the road by providing essential information within the line of sight and not at a centre console (from the handlebar) (Häuslschmid et al., 2018). We also decided to provide auditory and tactile cues through the head-mounted device to avoid complications of managing cues from multiple sources/locations.
Our early hazard notification concept
Here, we describe our bi-modal design concept, which consists of visual cues in combination with either auditory or tactile feedback. We considered notification timings of 9 s before a potential collision with a hazard. To minimise the distraction from the road associated with retrieving visual information from a handlebar-mounted smartphone/display, we provided the cues via a head-mounted device. We have mocked up a notification system using a Microsoft HoloLens 2 (3:2 aspect ratio, 2k resolution, 47 pixels per degree, diagonal FOV of 52°) and a self-built tactile headband (see Figure 1 a). With the notification concepts, participants received visual feedback in their direct line of sight on a HoloLens 2, supported by either auditory cues (from the HoloLens 2 speakers) or tactile cues (provided by the tactile headband). In Figure 1, we show the cyclists' view of the visual cue. A video demonstrating the AM concept with supporting auditory cues is available at osf.io/6mfj7/.
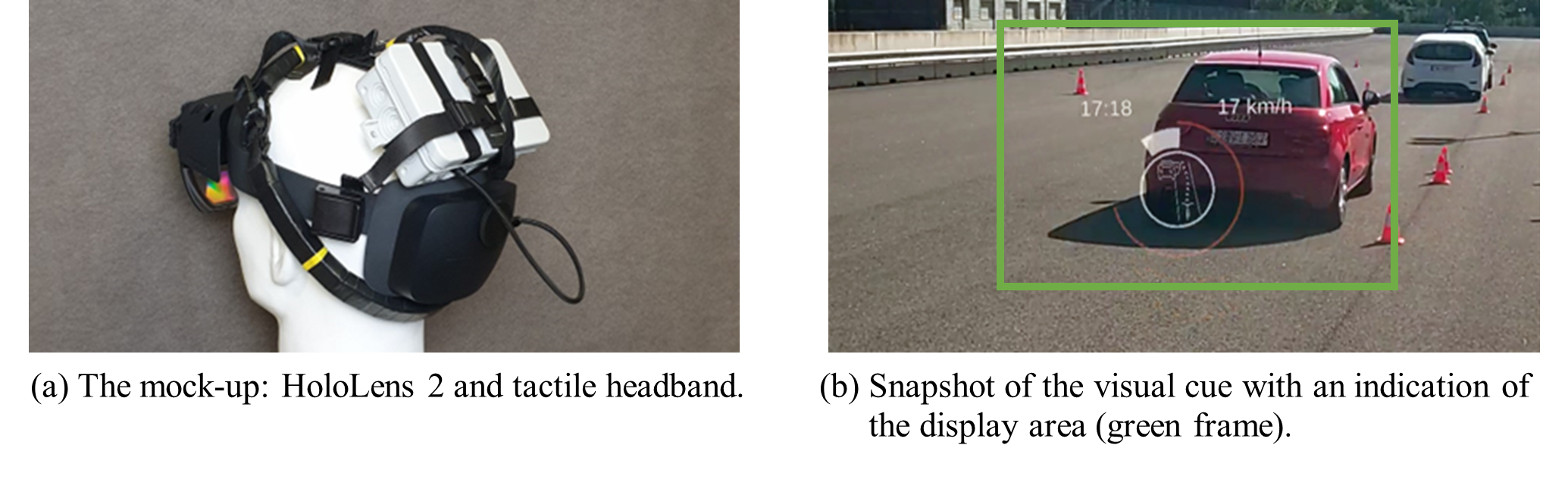
Design of the visual feedback
We used a screen-fixed 2D representation showing the information in the user’s direct line of sight (centre of the screen) to be visible independent of viewing direction. The direction of a hazard is indicated in a radar-like manner (circle segment), with a white icon depicting the kind of hazard in the centre. We anticipate that icons allow cyclists to quickly grasp which hazard to expect. A filling-up and colour-changing (green-yellow-dark orange) circle surrounding the radar shows the elapsed approximation time. We expected this filling circle to be easily visible in the cyclist's peripheral vision. If a hazard, such as an approaching vehicle, slows down during an active notification because it cannot overtake, the time display will remain at the lowest TTC previously recorded. This informs the cyclist that the hazard is still present, and that the alert is still active. When a hazard (is) passed, the visual fades out over a duration of 3 s. The visual was designed to be as transparent as possible by using lines thick enough to see the content but thin enough not to block the view. Visual cues were displayed at full brightness, but this was not sufficient outdoors. Thus, we placed a motorbike visor over the HoloLens 2’s projection area on the test track study. The visual representation was projected at a 2 m distance as recommended by Microsoft (2021) and took up about half of the horizontal screen size. Figure 2 shows the visual concept (a) and its temporal sequence (b).
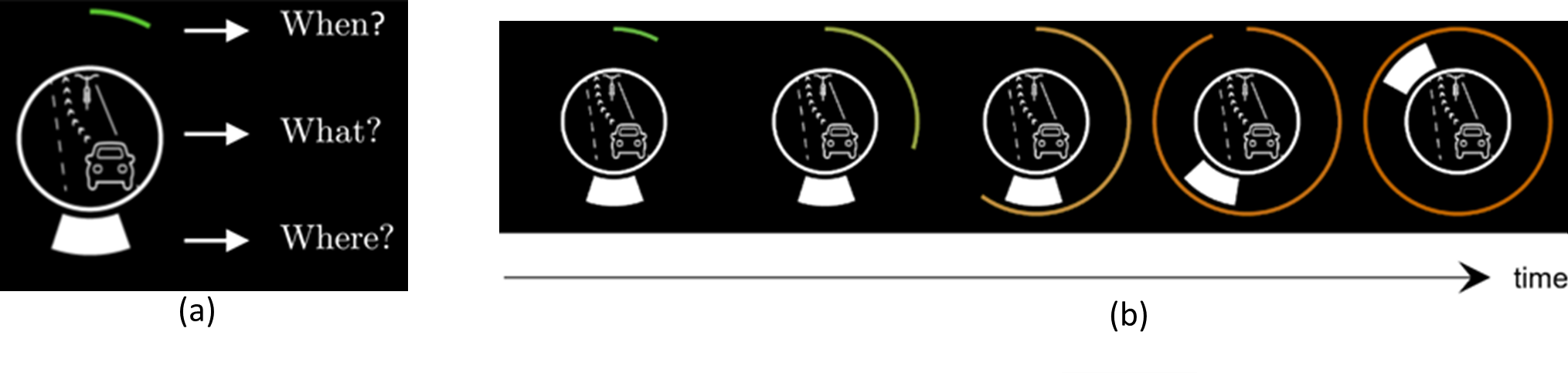
Design of the supporting auditory or tactile cues
The supplementary feedback was provided at distinct times (9, 6, and 3 s before being close to a hazard and 1 s after passing it, inspired by the concept of (von Sawitzky et al., 2022) to convey the temporal approach.
For the auditory feedback, the cues varied in pattern and length. The same sinusoidal tones as in Sawitzky et al. (2022) that we describe in Table 1 were used.
Message |
F 0 [Hz] |
dBFS* |
Duration |
Pattern** |
---|---|---|---|---|
incoming |
75 |
-72.3 |
0.70 |
ba-bling |
1st intermediate |
68 |
-49.2 |
0.20 |
be-boop |
2nd intermediate |
68 |
-50.2 |
0.45 |
be-boop-be-boop |
terminating |
51 |
-75.8 |
0.70 |
bada-dum |
*sounds played on a HoloLens 2 at a speaker volume of 60/100
**described with an onomatopoeic word
The tactile cues additionally indicated the direction in which the hazard is located (relative to the own heading), similar to the audio-tactile guidance method in narrow field of view AR displays by Marquardt et al. (2020) (but they indicated absolute direction by head orientation). For the provision of tactile cues on the head, Vo et al. (2021) suggested using four tactons in a helmet to mediate cardinal directions, while (Krauß et al., 2021) proposed a headband with 12 tactons evenly distributed for directional cues. They used a vibration frequency of 200 Hz, which they reported to be slightly higher than recommended by Oliveira et al. (2016), to make sure the cue is perceivable. As our headband had to be worn with the HoloLens2 (see Figure 1 a), space was limited, so we placed six tactons to indicate front right, right, back right, back left, left, and front left directions. We housed the tactons in a foam headband and thus had to use a higher frequency as the 32 Hz recommended by Myles and Kalb (2010) for tactons placed directly on the scalp. We used the maximum recommended frequency of 150 Hz (Myles & Kalb, 2010) and vibration durations of 200 ms. The tactile cues had the same duration, except for the terminat cue. Their intensity increased (up to maximum frequency) when getting closer to a hazard. The end cue made a circular motion around the head. A Wemos D1 Mini Wi-Fi module (with ESP8266 microcontroller) was used to control the headband. Straps with side-release buckles allowed adjusting it to different head sizes.
Evaluation with two user studies
We conducted two within subject design studies. Each focused on one kind of Hazard—either 'dooring' or ‘being overtaken’—and in both we investigated three types of Hazard Notification (within factor)—visual and auditory (V+A), visual and tactile (V+T) and no cues (baseline, B).
Our goal was to have participants experience these notification concepts while cycling under real-world conditions. However, due to ethical considerations, only the 'dooring' hazard was tested on a controlled test track, where safety measures allowed us to minimize the accident risk (see 4.2.2 for our safety measures). The study focusing on overtaking scenarios was conducted in a bicycle simulator as we deemed its conduction in a controlled test track study as being too dangerous. While investigating the notifications for both scenarios in both environments would have been ideal, we determined that testing overtaking situations on a test track was not feasible. Previous research on simulator validation (Sawitzky et al., 2023) demonstrated that human factors ratings related to the perception of notification concepts were valid in either absolute (e.g. user experience, perceived safety) or relative (e.g. workload) terms. Based on these findings, we anticipated that simulation and test track study results would yield statistically similar ratings. However, this way we were able to preserve some real-world applicability without compromising participant safety in situations involving moving vehicles.
Hypotheses and research scope
The primary objective was to assess (i) which of the two concepts is preferred for early hazard notification and (ii) whether these concepts are perceived as applicable to different hazards related to driver’s and their passengers’ incorrect behaviours. Our hypotheses were as follows:
-
H1: In combination with visual cues, supporting tactile cues are preferred over auditory cues, as they are not taxing the already occupied modalities (visual and auditory) any further and therefore capture the user's attention better.
-
H2: Early hazard notification is perceived applicable for both hazards we investigated—(i) ‘dooring’, and (ii) ‘being overtaken’, and both notifications are rated statistically equal (regarding user experience, perceived safety, workload and intuitiveness of hazard detection).
Technical setup and apparatus
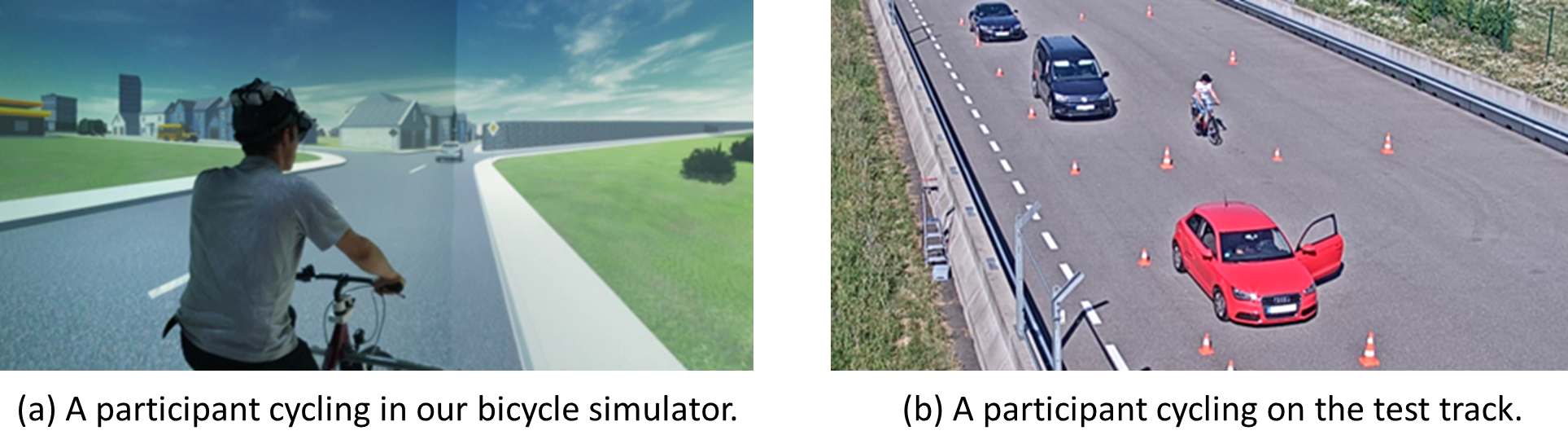
Study 1 (Bicycle simulator)
This study was conducted in a Cave Automatic Virtual Environment (CAVE), a four-sided (left, right, front, bottom), 3×3×3 m-sized projection room, in which we placed a low-entry bike in a roller trainer facing the right front corner (see Figure 3 a). This allowed participants to shoulder-check approaching traffic. Bike movement was calculated based on the handlebar angle (360° angular sensor) and cycling speed (Bluetooth IMU measuring angular velocity in °/s).
The simulation environment was created in MathWorks RoadRunner (Mathworks, 2022). It was set in a suburban area, on a two-lane track (width: 3 m, each) with sidewalks (1.2 m) and a length of about1.4 km. Vehicles drove at 50 km/h and slowed down to the cyclist's speed when oncoming traffic delayed the overtaking manoeuvre. Traffic sounds were simulated so that participants could hear the approach of vehicles. Cyclists were overtaken with a lateral distance of 1.5 m (distance between the vehicle side and the end of the cyclist's handlebar). Vehicles spawned dynamically based on the participant’s cycling speed to ensure that participants encounter similar situations. One overtaking vehicle and up to three oncoming vehicles were spawned for each overtaking task. Participants encountered five overtaking vehicles per ride.
Study 2 (Outdoor test track)
We used the same bike on the outdoor facility (see Figure 3 b). It was equipped with a GeneSys SP80 GNSS receiver to obtain differential GPS position and cycling speed (at 20 Hz). The track consisted of two lanes (width: 3 m, each), a parking lane (2.4 m), cycling (1.5 m), and pedestrian path (1.2 m). On the track, 5 vehicles were parked in the parking lane. For each scenario, the ride consisted of 12 laps á 350 m (about 4.2 km in total). Cyclists rode next to the parking lane about 1.4 km.
On the computer used for the simulation of the scenario (triggering of the notifications), the software also displayed the connectivity states of the devices. In case of a disconnect, the experimenter could then stop the participant and manually reconnect the service, e.g. restarting the dGPS tracker. This setup also enabled the experimenter to monitor the notification states and which vehicle the participant would be notified about in real-time so that the experimenter could signal when doors should be opened. Another person switched between the parked vehicles in a fixed order (per Hazard Notification) when the participant returned to the start of the lap and was not able to see this person. When no vehicle was to be occupied, this person hid behind the test track barrier. Potentially dangerous situations were reduced by considering occupied vehicles only in two-thirds (8/12) of the laps and opening vehicle doors only for half of them (4/12) and only when the cyclist was still further away. The experimenter was on the phone with the person switching vehicles and gave a signal when a door was to be opened by counting down from three when the cyclist was 9 s away from the vehicle. Thus, a door opened about 5 s prior to reaching it. Doors were only opened when deemed safe by the person in the vehicle. The lap was repeated if doors remained closed due to the safety measure. The ride ended when the experimenter stopped the participant.
Communication between components
Scenarios and hazard notifications were developed in Unity (Unity, 2024). The primary simulation communicated with CAVE and HoloLens 2 (Google Remote Procedure Calls and ProtoBuf, gRPC (The Linux Foundation, 2024)), headband (TCP), angle sensor (USB) and IMU (Bluetooth).
Measurements
Before the rides, we collect general participant information regarding demographics, cycling frequency and purpose, and affinity for technology interaction (Franke et al., 2019).
After each ride, we assessed user experience (hedonic and pragmatic quality) with the short version of the User Experience Questionnaire (Schrepp et al., 2017; UEQ team, 2024) and the intuitiveness of noticing and handling the potential hazards with the INTUI questionnaire (Ullrich & Diefenbach, 2010), 10 items addressing intuitiveness, gut feeling and effortlessness). Perceived safety (PS) was measured with the 13 items used in (von Sawitzky et al., 2022) study, addressing factors such as well-being, situational safety, and risk assessment. Workload impact was measured with the raw NASA task load index (Hart & Staveland, 1988). Each item was rated on a 7-point Likert scale.
After completing all rides, we asked participants to rate the Hazard Notification concepts (1: least, 3: most preferred). In the MR study, we assessed immersion (Slater et al., 1998), motion sickness susceptibility (Golding, 2006) and simulator sickness (Kennedy et al., 1993). In semi-structured interviews, we inquired about participants perception of the concepts, attitudes toward AMs, situations requiring support, and the effect of AMs. We also asked them about how they envision notifications for sequentially occurring hazards.
Data analysis
For the statistical analysis of the Likert scale participants’ ratings, we used JASP (JASP, 2024); for the reasoning why we used a parametric test for Likert scale data, see (Winter & Dodou, 2010). Analysis with a frequentist approach would require two individual tests, null hypothesis significance testing for differences and two one-sided tests for equivalence (Lakens, 2017). The Bayesian approach considers both the null (equivalence) and alternative (difference) hypotheses; it compares the relative evidence of the data's likelihood under the null or alternative (probability distribution) (Jarosz & Wiley, 2014). Due to multiple testing, the error rate accumulates in frequentist analysis. This is not the case with the Bayesian approach, as only one test is used to determine equivalence or difference. We applied a Bayesian Repeated Measure (RM) ANOVA with the fixed factors Hazard Notification (B, V + A, V + T) and Hazard (‘dooring’, ‘being overtaken’), including the participant number as a random factor to account for interindividual variance. We report model-averaged results (inclusion Bayes Factor, ). They are calculated by comparing the performance of models including this factor with models excluding it, which can be interpreted as the evidence in the data to include a model as a predictor of the data (Bergh et al., 2020). Post-hoc Bayesian t-tests were applied to further assess differences for which we reported Posterior Odds ( ), the respective Bayes Factors ( ) corrected for multiple testing. A .jasp file, including plots, data, and input options, is accessible at osf.io/6mfj7/.
The oral interview responses were categorized based on our interview questions and additional themes that emerged from the interviews.
Procedure
Both studies followed the same procedure. The participants were welcomed, briefed on the study scope and cyclist AMs. We emphasised their right to withdraw at any time, and particularly when feeling unwell (especially in the MR study due to simulator sickness). After signing an informed consent form and completing the pre-ride questionnaire, participants familiarised themselves with cycling in MR or on the test track. We then assisted them in putting on the HoloLens 2, tactile headband, and visor (test track only). Hazard Notifications were presented in randomized order, with explanations provided only for the current concept. These explanations included the type of feedback that would be provided on the ride (none, visual and tactile, visual or auditory). When a notification concept was tested, participants were briefed on the feedback’s timing, content, modalities, and presentation. The experimenter guided participants step-by-step through the notification so that they knew what feedback to expect. For this, different states of the visual notification were displayed on the HoloLens 2 and the supporting cues (auditory or tactile) were triggered. Participants then rode one lap along the track to experience the feedback in real time. We then asked if they could clearly perceive the visual, auditory, or tactile cues and if they understood the notification. If they did not perceive the cues clearly, we checked the correct fitting of the glasses, correct sound settings and issues with the headband, respectively, and participants re-did the familiarization ride. We also answered any further questions regarding the concept before continuing with the actual ride. The participants were then instructed to cycle along the track how they usually ride in traffic. Ride-related questions were completed after each ride, followed by final questions after all rides. The study concluded with semi-structured interview.
Study 1 (Bicycle simulator)
Participants cycled along the main street and encountered oncoming and approaching vehicles. Each ride took about 6 minutes, and the overall study 60 to 90 minutes to complete.
Study 2 (Outdoor test track)
Participants rode alternating on the street or cycling lane next to parked vehicles (indicated by arrows on the HUD). Each ride took 20 to 25 minutes, the overall study 120 to 140 minutes.
Participants
In total, we could recruit 53 participants (details in Table 2) via university mailing lists and by word of mouth. For the bike simulator study, we asked people who are sensitive to motion (e.g. on boats, in cars, on amusement rides, or in VR) not to participate in the study. Due to the use of Microsoft HoloLens 2 glasses during both studies, participants with large-frame glasses could not participate unless they wore contact lenses. There were no other inclusion or exclusion criteria. Participants were not compensated for their participation.
|
|
Study |
|
---|---|---|---|
|
|
‘dooring’ |
‘being overtaken’ |
Group size |
# |
21 |
32 |
Age |
M (SD) |
30.05 (4.74) |
28.50 (7.12) |
|
min-max |
21-39 |
20-51 |
Gender |
female |
4 |
14 |
|
male |
17 |
18 |
Cycling frequency |
daily |
4 |
11 |
|
several times a week |
7 |
5 |
|
once a week |
2 |
7 |
|
sporadically |
8 |
9 |
Cycling purpose |
commute |
12 |
12 |
|
free time |
16 |
9 |
|
exercise |
6 |
11 |
Affinity to technology interaction |
M (SD) |
4.78 (0.64) |
4.59 (0.61) |
Results
Our findings are based on our participants' responses. Due to technical problems, ride-related questionnaire responses of three (’being overtaken’) and one (‘dooring’) participant had to be omitted, but we included their interview responses in our report.
Ride-related responses
We conducted a statistical analysis of the obtained questionnaire data using Bayesian ANOVA. Figure 4 shows the descriptive plots. The data points indicate the mean (and 95% CI) of our participants ratings (y-axis, 7-point Likert scale, 1—low, 7—high rating) for the measured constructs for each Hazard Notification (x-axis) where unfilled dots indicate the ratings of the group that experience ‘being overtaken’ and filled dots the ratings of those that experienced ‘dooring’ hazards.
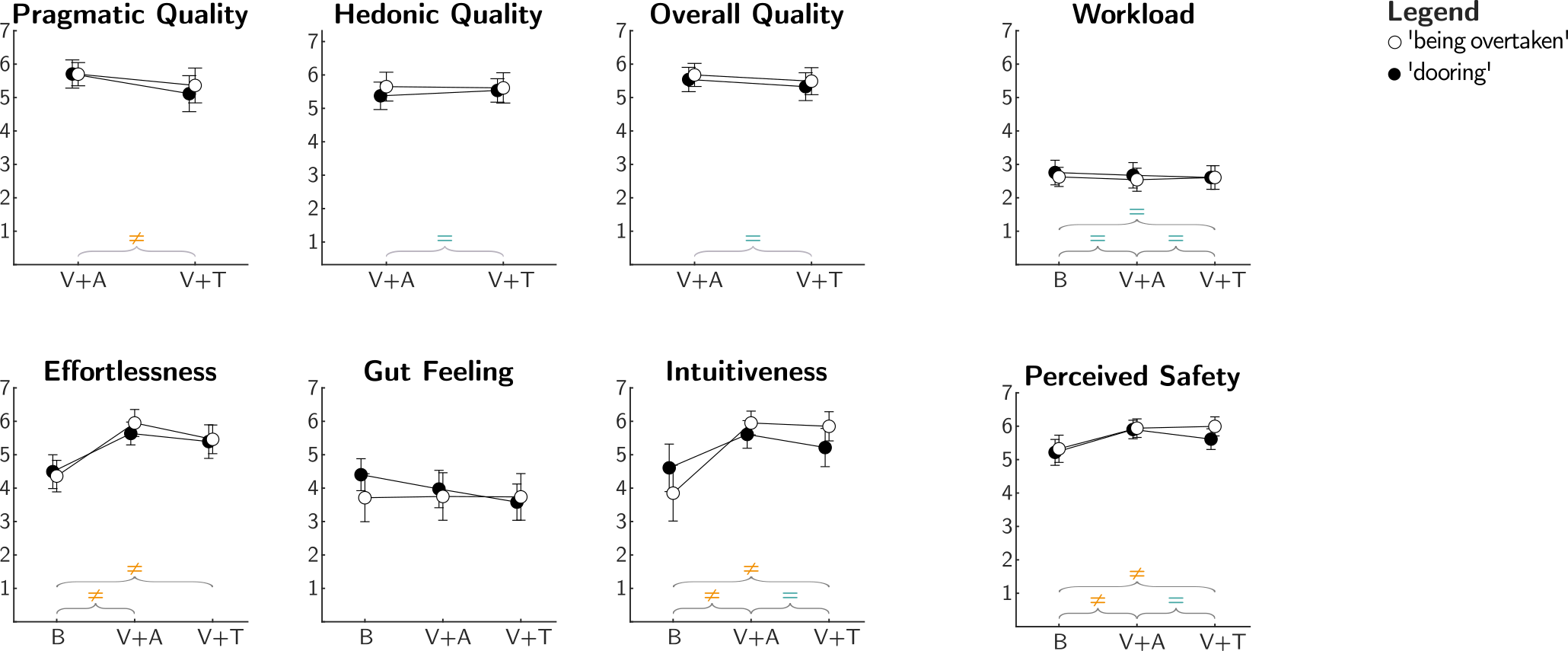
Table 3 shows the test statistics for our analysis regarding the effects of the factors Hazard Notification, Hazard and their interaction.
|
Bayesian ANOVA |
|||
---|---|---|---|---|
Measurements |
Effects |
|
Conclusion |
Evidence |
Pragmatic quality |
Notification |
2.671 |
different |
anecdotal |
Hazard |
0.265 |
equal |
moderate |
|
Notification × Hazard |
0.260 |
equal |
moderate |
|
Hedonic quality |
Notification |
0.189 |
equal |
moderate |
Hazard |
0.321 |
equal |
moderate |
|
Notification × Hazard |
0.093 |
equal |
strong |
|
Overall quality |
Notification |
0.406 |
equal |
anecdotal |
Hazard |
0.282 |
equal |
moderate |
|
Notification × Hazard |
0.115 |
equal |
moderate |
|
Effortlessness |
Notification |
3.608⋅107 |
different |
extreme |
Hazard |
0.216 |
equal |
moderate |
|
Notification × Hazard |
0.165 |
equal |
moderate |
|
Gut feeling |
Notification |
0.471 |
equal |
anecdotal |
Hazard |
0.330 |
equal |
moderate |
|
Notification × Hazard |
0.285 |
equal |
moderate |
|
Intuitiveness |
Notification |
29564.498 |
different |
extreme |
Hazard |
0.418 |
equal |
anecdotal |
|
Notification × Hazard |
1.311 |
different |
anecdotal |
|
Workload |
Notification |
0.060 |
equal |
strong |
Hazard |
0.276 |
equal |
moderate |
|
Notification × Hazard |
0.011 |
equal |
strong |
|
Perceived safety |
Notification |
8971.533 |
different |
extreme |
Hazard type |
0.372 |
equal |
anecdotal |
|
Notification × Hazard |
0.320 |
equal |
moderate |
Effect of Hazard notification
From a first visual inspection of the data presented in Figure 4, we could derive that user experience (pragmatic, hedonic, and overall quality) was rated rather high for both notification concepts. Workload ratings for rides with and without hazard notification appear similarly low. Further, effortlessness and intuitiveness of noticing a hazard were rated rather higher for rides with a system than unassisted rides (middle range). Ratings for gut feeling were in the middle range. Perceived safety for unassisted rides was relatively high but the rating was slightly higher for assisted rides.
For the factor Hazard Notification, there is evidence for equivalence, i.e. that it did not impact the ratings on hedonic quality, and workload, but the evidence was only anecdotal for overall quality and gut feeling. For the other measurements we found evidence for difference. Thus, we applied post-hoc Bayesian t-tests to gain further insights. For effortlessness ratings the tests provide extreme evidence that the ratings for no feedback (B) were lower than with V+A (PO = 508555.112), also when compared with V + T (PO = 169.528). There is anecdotal evidence that the ratings for V + T were equal to V + A (PO = 0.425). For intuitiveness, there is extreme evidence that V + A is rated higher than B (PO = 2217.207) and very strong evidence that V + T is rated higher than B (PO = 36.839). Ratings for V + A and V + T showed moderate evidence for equivalence (PO = 0.228). For perceived safety, the ratings for V + A (PO = 92.397, very strong evidence) and V + T (PO = 5.366, moderate evidence) were higher as for B. Also, V + A and V + T were rated equally with moderate evidence (PO = 0.199).
Effect of Hazard
For the factor Hazard, there was evidence for equivalence for all measurements. However, for intuitiveness and perceived safety this effect was only anecdotal.
Effect of Hazard notification × Hazard
Except for anecdotal evidence for difference of intuitiveness ratings, there was evidence for equivalence for the other measurements and the interaction effect did not impact the ratings.
Post-study responses
Of our participants, (‘dooring’: 13, ‘being overtaken’: 16) preferred V + A, 31% (7 and 9) V + T, and 13% (1 and 6) no feedback (B). In the simulation, participants had a moderately strong sense of presence (M = 4.64, SD = 0.79). They showed a low level of simulator sickness (M = 30.39, SD = 26.34; maximum: 235.62): nausea (M = 28.92, SD = 31.64), oculomotor (M = 21.79, SD = 18.34), disorientation (M = 30.89, SD = 34.23). The MSSQ scores were also low with an average score of 12.14 (SD = 10.60) of maximum 54 possible.
Interview responses
Below, we list an overview of our participants’ responses from the semi-structured interview. The absolute number of participants who stated certain aspects are indicated in parentheses.
Effect of AMs
Additional support was perceived as always helpful for VRUs (5). About 67% of participants found AMs valuable and helpful in enhancing awareness of potentially dangerous situations (36). AMs would improve their situational awareness by being more alert (15) and increase their sense of safety (8). Notifications further facilitated detecting approaching vehicles, requiring less concentration (5), and allowing time for preparing to act (12). Some individuals expressed reluctance to use AMs (6). They did not want to be disturbed (1), distracted (1), did not see an added value (1), or stated to be capable of navigating traffic safely on their own (3).
Perception of AMs
Timing. Most participants found receiving notification 9 s before encountering a hazard suitable (31, about 85%); however, some perceived the notification as too early but could imagine being notified 5–6 s ahead of a potential collision (12, about 22%).
Sensory perception of cues. After the familiarization ride, we asked participants, whether the provided cues were perceptible and clear. All responded in the affirmative, apart from one participant in the simulator study with very curly hair, who perceived the tactile feedback but would have appreciated a stronger feedback intensity.
Visual cues. Icons were perceived as an effective way to indicate potential hazards (37). For some participants, the visual cue was distracting, confusing due to too many factors displayed, or hard to focus on (5). For others, the direction indicator clashed with the time indicator as they moved in the same direction (3). For some (3), the visual blocked their view.
Auditory cues. Compared to tactile cues, they were more comfortable (9), increased attentiveness (4), were more intuitive (4), more unambiguous (2) and easier to notice and understand (3). Further, they were familiar from games (1) or navigation (2). However, some participants felt detached from the situation (3) or distracted (4) or found it annoying or uncomfortable (2). Particularly in noisy environments (5) or when listening to music (2) sounds could be hard to notice. The initial sound was startling (5), or disturbing (3). Multiple occurrences of auditory cues could reduce attention paid to the AMs (1).
Tactile cues. Compared to auditory cues, tactile cues were perceived as more comfortable (9), like a nudge (1), not distracting (2), better comprehensible (4), less intrusive (1), more subtle (3) and made more aware (3). Tactile cues were easier to notice (also in noisy environments) (2) and allowed for easier hazard localization (3). However, they were also perceived as confusing (5), ambiguous (4), strange (2), distracting (4), irritating (9), startling (1), or too intimate (1). Some found the end notification too strong (11), or vibrations felt uncomfortable (2). A prolonged use could induce headaches (1).
Proposed adjustments
Visual cues. Some participants liked the presentation of the visual cues and saw no need for further improvement (23). For some only sounds or vibrations would suffice to be more alert (5). It was suggested to place the visual off-centre (6) or on the screen borders pointing in the direction of the hazard (2). Position, scale, and transparency should be configurable (5). Further, content visibility could be increased with thicker lines (5). The icon should indicate vehicle types (3). To better distinguish the direction and time indications, a horizontal progress bar could be used (2). The time indicator could be replaced if the color-coding is transferred to the direction indicator (3). Red-green colour blindness should be considered (1). Additionally, it's transparency could be reduced after a few second to be less present in the field of view (1).
Supporting cues frequency. Auditory cue volume should be adjustable (5). The end vibration could be replaced with a sound (1) or two short vibrations (2). Some stated they would not need an end notification (13). Others would only require an initial indication to be made aware of the notification (6). It was suggested to provide the vibration away from the head on the handlebar (1) or through a bracelet (1). Vibrations should be distinct, as the tones were (1), and their intensity should be adjustable for varying hair density and improved perception (5).
Design ideas for presenting repeating occurrences
Visual indicators. Participants suggested indicating multiple occurrences near the hazard icon, either signalling the number of vehicles (14) or that there will be more encounters (5). The icon could display multiple vehicles (6), or multiple instances of the visual could be stacked (1). Alternatively, the direction indicator could mimic browser tabs (4) or stacked from the inner to the outer area (4). They could also point toward the direction the hazard will be the closest to the cyclist (4) or their initial direction (2). The time indicator could either disappear after the occurrence of the first hazard (3), stay filled (5), update for subsequent vehicles (4), or overlay a second indicator (9). Also, time information could be coded into the direction indicators (1).
Tactile and auditory indicators. Our participants could imagine continuing the same cues as for the intermediate steps and a terminating cue (9) or only using start and end notifications (2).
What to notify about
AMs should inform about potential dooring situations (19), about approaching cars, at intersections during crossing and left or right turns (19), about vehicles taking off (parking space or driveway) (5) and when cyclists are in a blind spot (2), or nearing accident black spots (1). It would be helpful to notify about e-vehicles (7), e-scooters or e-bikes (3) and other cyclists in their vicinity, e.g. when they are behind them and could overtake (4), about ghost riders (3) or when there is a high number of cyclists (2), crossing pedestrians/children (14). Some want to be notified about ground conditions, e.g. road surface, grooves, potholes (6). It was also proposed to include navigation (3), weather forecast (2) and traffic lights (5) notifications.
AM support could be unnecessary when cycling on fields/in forests (5), when encountering obvious dangers (4), in calm areas (2), in very crowded areas (2) or on distinct and broad cycling lanes (3). It would likely be impossible to notify about unpredictable situations, such as small objects on the roads or the movement of children, pedestrians, or animals (3).
Challenges
Participants highlighted the necessity of a longer adaptation period to grasp the notification system fully (17). Some participants noticed a learning effect over time (6). However, some participants reported concerns about over-reliance on AMs, as they quickly relied on the AMs and paid less attention to their environment (15), which could become dangerous (1). One participant suggested shifting the visual cue to the side to avoid solely focusing on it.
Additional remarks
AMs would benefit those wanting to be safe in traffic (1) or children and older people (5). They would also be beneficial to cyclists listening to music by either using a V + T concept (2) or integrating V + A and overlaying the music with cues (2). V + T could be particularly useful for hard-of-hearing cyclists (1). The notification device should be lightweight and comfortable (8), preferably integrated into helmets (3) or glasses (8). Due to vibrations (ground, cyclist movement), the displayed content bobbed slightly up and down; also, the glasses may slip from their initial position, reducing the content visibility (1). The system should be configurable (4), and also notify about immediate hazards (3). When encountering hazards simultaneously, the notifications should not be overwhelming (6); prioritizing hazards could help in such a case (1).
Discussion
We assessed two bi-modal notification concepts for early hazard notification for cyclists through AMs (visual and auditory icon, visual and tactile cue) in two user studies focusing each on a single hazard (‘dooring’, N = 21, or ‘being overtaken’ by a vehicle, N = 32). We aimed to explore the concepts’ applicability to different hazards that cyclists may encounter with motorised vehicles and to further gain insights on their impact on the cyclist and the notification design.
Preference: tactile vs. auditory supporting cues (H1)
Contrary to our expectations, the overall most preferred supporting cue for AMs was auditory and not tactile, as hypothesised, so we cannot accept hypothesis H1. Some participants stated they felt they would not need additional support, saw no added value, or felt that feedback was merely a distraction. This uneven distribution of preferences could be the reason for the higher ratings of pragmatic quality for V + A (compared to V+T); users are likely to be familiar with auditory feedback (e.g. navigation cues or in-vehicle warnings) and less familiar with tactile cues. Some participants associated certain characteristics only with their preferred concept type, e.g. more convenient than the other, not distracting, and easier to understand. Almost a third of the participants found the Hazard Notification with tactile feedback more pleasant, subtle, easier to understand and less intrusive than auditory feedback. As the high ratings for the hedonic quality and perceived safety were evidently unaffected by the modality of the supporting cue, we assume that both types were useful. The preference distribution is comparable with that reported by Erdei et al. (2021), who considered uni-modal notifications provided from the handlebars. The location the cues are originating from seems not to affect the distribution.
Feasibility of a generalizable awareness message concept (H2)
Most of our participants thought that AMs will be a helpful support for cyclists. Our data provided convincing evidence that participants’ ratings were the same (user experience, intuitiveness, workload) or rather similar (perceived safety) for both Hazards. While these results support hypothesis H2 (similar rating for each Hazard), this similarity does not confirm a positive impact of the AM concepts. Our further analysis revealed that the use of AMs (compared to riding with no feedback) had a positive effect on the effortlessness and intuitiveness of recognising potential hazards. These results are less surprising, as the system informs about the presence of a hazard ahead of time, thus increasing situational awareness. Prior knowledge of potential hazards and the opportunity to prepare countermeasures likely contributed to increased perceived safety. Consistent with the findings of Springer-Teumer et al. (2023) and (Sawitzky et al., 2022) the workload was the same as for unassisted cycling. Thus, perceiving and processing of the information provided did not add to the workload.
Notification design
Overall, participants were satisfied with the way the notifications and the information provided (type of hazard, its relative direction, elapsed approximation time); only few participants stated that they would not need visual cues, probably because only one hazard was considered in the study. Knowledge of the type of hazard was essential for about two thirds of our participants. The icons were perceived as intuitive indicators that helped them identify potential hazards. 23 participants would want to use the visuals as shown in this study. Only a few of our participants found the visuals distracting, in contrast to the findings of related work where visual feedback was reported as distracting from the cycling task (Erdei et al., 2021; Matviienko et al., 2019). This discrepancy may be influenced by the device used to provide the visual cues. The location of visual cues appears to be a crucial factor. In our study, participants could obtain information in their peripheral vision or briefly focus on the visual cue to see it clearly. They did not have to tilt their head to receive visual cues from the handlebar. Moving cues from the handlebars to the head may enhance the perceptibility of auditory and tactile cues. Our participants did not report that either supporting cue was hard to perceive.
Design challenges
The intended purpose of AMs is to inform cyclists in advance of potential hazards. The underlying algorithm can only detect foreseeable potential hazards. It is unlikely that it will predict spontaneous hazards. For example, dooring can happen when a vehicle stops briefly at the roadside, and someone exits (Johnson et al., 2013). Cyclists might still face hazards that the system cannot foresee, even if it can provide notification about this specific kind of hazard.
Further, in each user study, we focused only on one hazard and only one incident at a time. However, it is likely that multiple hazards occur in close sequence or also simultaneously. One participant proposed that it may be required to prioritise some hazards over others. Being overtaken and dooring situations sometimes overlap. In such cases, either one type of hazard must be prioritised, or both hazards must be indicated. Both approaches come with their own set of advantages and disadvantages. When prioritising, the most urgent/relevant hazard could be specified, but other hazards are disregarded. The indication of multiple simultaneous hazards at once provides a more comprehensive overview of potential hazards but could also lead to distraction and overload as a lot of information needs to be displayed.
Challenges for the use(rs) of AMs
One major concern stated by our participants was that users may become overly reliant on these notifications, an issue also raised by Matviienko et al. (2022); Springer-Teumer et al. (2023) and (von Sawitzky et al., 2022), though not reported by their participants. This problem of overreliance is common to support systems, e.g. advanced driver assistant systems (Geels-Blair et al., 2013; Wintersberger et al., 2019). Further research should focus on preventing hazard blindness to (other) hazards due to overreliance and ensuring that users to stay alert. Further, some of our participants expressed a desire for a longer familiarisation phase with the notification. The potential of such a phase to help cyclists understand and learn the correct use of AMs and their limitations needs to be further explored in future work.
Design recommendations for early hazard notifications for cyclists
We derived the following recommendations for the design of hazard notification that are provided on head-mounted systems, such as smart cycling glasses. The following list is not a comprehensive set of recommendations, but it reflects those derived from the research presented in this paper.
Recommandation 1. Sufficient notification time should be allocated to allow users to notice and understand the notification, to identify the hazard, to consider which countermeasure is appropriate, and act if necessary.
While many of our participants found a timing of 9 s ahead to be adequate and stated that this time frame allowed them to prepare to react to a hazard, some would have preferred a later indication at 5-6 s prior. A timing of about 6 s prior before a potential collision (Gold et al., 2013) may still allow sufficient time for situation processing and reaction. Although further research is needed to examine the impact of shorter notification times, we assume that too short time frames could lead to overwhelm. Users may not be able to process the provided information and also react to the potential threat. Other studies on cyclist hazard notifications (Engbers et al., 2018; Oczko et al., 2020; Strohaeker et al., 2022) considered shorter time frames but provided less detailed information about the situation. For a system designed to support multiple kinds of hazards arising from interactions with motorists, we anticipate that providing more detailed information is crucial to enable fast and intuitive interpretation. Therefore, we assume that ensuring sufficient time for processing and acting is essential.
Recomandation 2. Unambiguous icons or symbols should convey information about the kind of hazard the notification addresses.
Many participants found the icons indicating the type of hazard to be an effective way to communicate potential dangers. We assume that using distinct icons can minimize the misinterpretation of hazards, further users likely become familiar with their meanings quickly.
Recommandation 3. Bi-modal cues should be used. While relevant information should be provided visually, another modality should be used to guide the cyclist without requiring them to focus on the visual content. Here, the peripheral field of view should also be utilized.
Both bi-modal concepts that our participants experienced were perceived as useful. Few participants indicated that guiding cues alone would be sufficient for notification. However, we suspect that it may be difficult to identify hazards, when visual information about the hazard is not indicated.
Recommandation 4. The used head-mounted device should be comfortable to wear.
Comfort is crucial for user acceptance and long-term use of the notification device. Cyclists should not be distracted or irritated by wearing the device during their rides.
Recommandation 5. The notifications should be customizable in a predefined extent.
Participants expressed a desire to customise AM presentation according to their preference, such as adjusting volume, intensity, or when notifications are issued. Allowing some customization ensures that cyclists can adjust the notifications to their needs. This customization also includes that the type of additional feedback modality (auditory or tactile) can be selected by the user, as some of our participants stated to rather not use the support if it is not their preferred feedback type.
Parallels to existing HMI guidelines. Naujoks et al. (2019) proposed a checklist of 20 design guidelines for human-machine interfaces (HMIs) in automated vehicles, taking into account existing guidelines, design recommendation and best practices in this context. While some of these guidelines are not relevant to notifications for cyclists—being more focused on the functionalities of automated vehicles, such as operation principles or system mode indication—two closely resemble our design recommendations for cyclist hazard notifications. For example, guideline #9 suggests using ‘[c]ommonly accepted or standardized symbols [(such as pictograms)] […] to communicate the automation mode’. Similarly, our recommendation #2 suggests using icons to convey the hazard type. Additionally, guideline #6 advises that ‘[t]ime-critical interactions with the system should not require continuous attention’. Although this guideline differs slightly from our recommendation #3 that guiding cues should be used to allow cyclist to maintain their visual focus on the road, both emphasize minimizing the need for frequent interaction with the system.
We argue that some of the other guidelines could also be considered as recommendations for cyclist hazard notifications, e.g. guideline #16 suggests ‘[a]uditory output should raise the attention of the driver without startling her/him or causing pain’. Relating this guideline to our proposed notification, this advice would apply to both supporting cues (auditory, and tactile) that we investigated. While not all of (Naujoks et al., 2019)'s guidelines are directly applicable to cyclist hazard notifications, they offer valuable reference points for future research aimed at developing guidelines for cyclist notification on smart glasses.
Reflections on the ecological validity of early hazard notifications for cyclists
While our research focused primarily on the general design and applicability of such notifications for two specific hazards, actual traffic situations place additional demands on the requirements of such a system. Given the inherent complexity of traffic, both our study and most of the related works on hazard notifications have only investigated relatively simple scenario as a proof-of-concept for their design. In the following, we discuss the ecological validity of the proposed early hazard notification concept and explore required extensions as well as potential challenges that need to be addressed in their design. We identified two additional situations that the system must be capable of handling, which have not been addressed in this work:
The motorist or cyclists makes a turn or changes lanes during an active notification. In our current approach, notifications terminate only after a potentially hazardous situation has been resolved. However, in real world scenarios, notifications may need to be terminated prematurely if the user or vehicle change course, and the hazard no longer persists. We assume that the challenge here likely lies in accurately detecting a change in course.
Multiple hazards are present simultaneously/ subsequently. In this work, our research concentrated solely on situations where cyclist encounter a single hazard at a time, rather than multiple hazards. In real traffic conditions, encountering multiple occurrences of hazards is quite likely. From our interviews, we have gathered our participants’ suggestions on how to notify cyclists about multiple hazards. They could be used as a starting point for further research in this regard. A major challenge we suspect in addressing multiple and potentially simultaneous hazards is the risk of information overload, which could overwhelm and distract cyclists.
From this reflection we can derive two additional aspects of early cyclist hazard notification systems, which should be investigated further in future work: (i) the prematurely termination of notifications; (ii) the indication of multiple hazards.
Limitations
Our notification concept is primarily based on previous work implementing support systems and/or evaluating hazard notification for cyclists. Participants only encountered single occurrences of one hazard type. Our user studies, conducted with a single cyclist and a limited number of other road users, reflect only low-traffic conditions. For ethical reasons, this study was conducted in controlled, staged scenarios on a test track and in simulation rather than in real traffic. In addition, the concepts in both studies were evaluated with a small sample size, consisting primarily of students and university staff. As a result, the samples are not fully representative of the broader cycling population in Germany, which limits the generalizability of our findings. Situational awareness was not measured. Further, the investigated hazard types and simulation environment were not independent, as different hazards were experienced in different test environments.
Future work
Since visual and auditory, but also visual and tactile cues seem feasible for cyclist hazard notification, and about a fourth of our participants would only use their preferred cues, both combinations should be further explored. While our findings suggest their feasibility for both hazard types studied, further investigation is needed for different hazard types (such as those proposed in 5.3.5), multiple occurrences, and notification conflicts. Further, strategies to facilitate user learning and avoid overreliance on support systems need to be addressed. In order to extend the notification concept to address simultaneous hazards, researchers could consider the ideas suggested by our participants (detailed in 5.3.4). While this study has only focussed on cyclist-directed notification, bi-directional feedback should be considered to also notify other road users involved of the potential hazard.
Conclusions
Vulnerable road users (VRUs), such as cyclists, need to remain vigilant in traffic as they cannot rely solely on the attention of other road users to ensure their safety, but detecting hazards, especially 'out of sight' hazards, is often a challenge. To address this issue, we proposed early hazard notifications delivered at head level via smart glasses to assist cyclists by making them aware of potential hazards. We compared notification concepts delivered through smart glasses (visual cues) in combination with either auditory (sounds emitted from the glasses' speakers) or tactile (from a tactile headband) cues in two user studies, each investigating a single hazard situation—'dooring' and 'being overtaken'. The key findings of this work are:
-
Support for different hazards could be inferred for both cues. Regardless of the hazard, participants perceived the concepts similarly. Both notification concepts (visual and auditory, visual and tactile) had a positive effect on effortlessness, intuitiveness and perceived safety during cycling and were rated higher than receiving no feedback; the overall workload was comparable to rides without feedback. Participants felt well-supported in both scenarios and saw potential for these awareness messages for other hazardous situations while cycling. However, further research is required to determine the concept’s applicability for other hazards.
-
Feedback preferences show a tendency towards visual and auditory cues, but a significant proportion of participants preferred visual and tactile cues. Notably, some participants would choose no feedback over their less preferred assistive cue (auditory or tactile). Further research should consider both bi-modal combinations.
-
Based on our requirements for the notification design and the results of our study, we provide design recommendations for the design of early hazard notifications for head-worn devices.
Given that VRUs are often at risk of being involved in vehicle accidents, equipping them with tools to better anticipate hazards could reduce their risk of such incidents. Early hazard notifications, in particular, could be a promising option for achieving this by giving cyclists the chance for proactive intervention.
Acknowledgement
We would like to thank Jason Bilevitz, who was involved in designing the hazard notification concept and conducting the ‘being overtaken’ study as part of his bachelor's thesis. Special thanks go to Jason Bilevitz and Michael Weinzierl for their active support during the conduction of the test track study. Our thanks also go to the team at the CARISSMA outdoor facility for their help and advice during set up, testing, and conduction of the test track study. We would also like to thank our reviewers for their valuable insights and constructive feedback, which contributed to refining and strengthening this article. We have applied the FLAE approach for the sequence of authors.
An early version of this paper was presented at the 11th International Cycling Safety Conference, held in the Hague, the Netherlands, on 15–17 November 2023.
Declaration of competing interests
There are no competing interests.
Ethics statement
Study participation was following the ethical guidelines of the Declaration of Helsinki (WMA, 2013). Participation was voluntary. Participants were obliged to provide their written informed consent. They could withdraw from the study at any time and leave comments or feedback. As there was no ethical review board at our university at the time of study planning, implementation and conduction, we developed our own measures. To ensure the safety of the participants, but also to allow the participants to experience dooring hazards in the test track study, we applied the safety measures described in 4.2.2. In addition, we ensured data privacy by not storing the IP addresses of the devices used to complete the questionnaire and by deleting the audio files and their backup copies after transcription, in accordance with Article 17(2) of the GDPR (EU, 2018).
Funding
This work is supported under the ‘Innovative Hochschule’ program of the German Federal Ministry of Education and Research (BMBF), Grant No. 03IHS109A (MenschINBewegung) and by the German Federal Ministry of Transport and Digital Infrastructure (BMVI) within the Automated and Connected Driving funding program under Grant No. 01MM20012J (SAVeNoW).
CRediT contribution statement
Tamara von Sawitzky: Conceptualization, Data curation, Formal analysis, Investigation, Methodology, Project administration, Software, Validation, Visualization, Writing—original draft.
Andreas Löcken: Conceptualization, Methodology, Validation, Writing—original draft.
Thomas Grauschopf: Conceptualization, Funding acquisition, Resources, Supervision, Writing—review & editing.
Andreas Riener: Conceptualization, Resources, Supervision, Writing—review & editing.